Keynote speaker
Cameron Ghalambor
Does phenotypic plasticity facilitate or constrain adaptive evolution?
Abstract
A fundamental, but unresolved, question in evolutionary biology is how patterns of plasticity observed within a generation influence evolutionary changes across generations. Theoretical models examining the relationship between plasticity and evolution have made conflicting predictions, with some models arguing that plasticity constrains evolutionary responses, while others argue for plasticity having a facilitating role. Few empirical studies have been able to test these ideas, because the initial stages of adaptive divergence are not observed. Adaptation to new environments, such as those encountered during colonization events or those generated by human activities, are provide an opportunity to test the relationship between initially plastic responses and subsequent evolutionary change. We have experimentally transplanted Trinidadian guppies (Poecilia reticulata) from streams where they are adapted to living with cichlid predators to cichlid free streams, and tested how plasticity in the source population relates to evolutionary change in the transplanted populations. We have measured a suite of traits, including gene expression, body shape, growth rate, and metabolism. We find evidence for both adaptive and non-adaptive plastic responses in the source population and rapid evolution of traits and their plasticity in the experimental population. This plasticity influences evolution by altering the strength of selection and patterns of covariance among traits. For example, traits exhibiting non-adaptive plasticity (i.e. a plastic response in the opposite direction favored by selection) diverged from the source population more rapidly, suggesting they are subject to stronger directional selection and evolve more rapidly compared to traits exhibiting adaptive plasticity.
Presenters
karin burghardt
The role of plastic plant defense strategies in determining landscape level variability in
ecosystem processes
Abstract
During old-field succession, homogenous agricultural soils transition into spatially and temporally variable field soils. This variation is typically attributed to the composition of underlying bedrock and plant biomass production. However, phenotypic plasticity within dominant plant species may play an overlooked role in creating soil heterogeneity. Theoretically, herbivory across nutrient gradients could generate plasticity-mediated changes in the nutrient cycling, through plastic changes in plant defenses, resulting mosaics of ecosystem process rates across a field. I report on how phenotypic plasticity in defense influences soil nutrients, resolved using a combination of field surveys, lab assays, greenhouse pot experiments, and a three-year raised bed experiment. Grasshopper feeding causes clones of a dominant oldfield plant goldenrod to exhibit tolerance at low nutrient levels and induced resistance at high nutrient levels. In turn, chemical and structural changes in high nutrient “defended” plant litter result in slower microbial decomposition and changes to a host of ecosystem processes. Thus, herbivores may indirectly shape the nutrient environment experienced by the plant, potentially creating eco-evolutionary feedbacks that either accelerate or decelerate nutrient cycling locally. By highlighting intraspecific drivers of spatiotemporal variability, this work provides empirical support to the theory emphasizing the importance of plasticity-generated trait variability to ecological processes.
Oana Carja
The evolutionary advantage of phenotypic plasticity
Abstract
Environmental variation is commonplace, but unpredictable. Phenotypic plasticity is an evolutionary driving force in diverse biological processes, including the adaptive immune system, the development of neoplasms, and the persistence of pathogens despite drug pressure. It is essential, therefore, to understand the evolutionary advantage of alleles that confer the ability to express a range of phenotypes. This type of evolutionary bet-hedging need not confer a direct benefit to a single individual, but it may increase the chance of long-term survival of a lineage. I will discuss several population-genetic models that explore how partly heritable phenotypic variability influences evolutionary dynamics in populations. For example, populations that encounter a deleterious environment can sometimes avoid extinction by rapid evolutionary adaptation. I will show that the advantage of such a plastic mutation depends on the degree of phenotypic heritability between generations, called phenotypic memory. Moreover, the probability of population persistence depends nonmonotonically on phenotypic memory: some heritability can help avert extinction, but too much heritability removes any benefit of phenotypic plasticity. I will discuss the implications of these results in the context of therapies designed to eradicate populations of pathogens or aberrant cellular lineages.
Yuheng Huang
Experimental evolution of gene expression and plasticity in alternative selective regimes
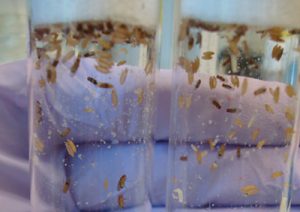
Abstract
Phenotypic plasticity is presumed to evolve adaptively in response to environmental heterogeneity but little is known of how this happens. As environmentally induced gene expression changes are essential for phenotypic plasticity, we measured the transcriptome in a cadmium-enriched diet and a salt-enriched diet for experimental populations of Drosophila melanogaster that evolved for ~130 generations in one of four selective regimes: two constant regimes maintained in either cadmium or salt diets and two heterogeneous regimes that varied either temporally or spatially between the two diets. For populations evolving in constant regimes, we find a strong signature of counter-gradient evolution; the evolved expression differences between populations adapted to alternative diets is opposite to the plastic response of the ancestral population that is naïve to both diets. Based on expression patterns in the ancestral populations, we identify a set of genes that were expected to be selectively increased in plasticity in heterogeneous regimes and we find the expected pattern. In contrast, a set of genes where we predicted reduced plasticity did not follow expectation. Nonetheless, both gene sets showed a pattern consistent with adaptive expression evolution in heterogeneous regimes, highlighting the difference between observing “optimal” plasticity and improvements in environment-specific expression.
Holly Moeller
Trade, borrow, or steal: how acquired metabolism confers phenotypic plasticity
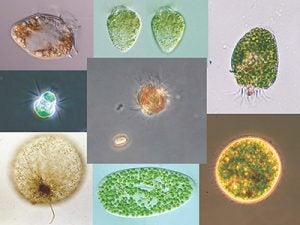
Abstract
An organism’s ecological niche is fundamentally constrained by its metabolism, which
determines its ability to take up and transform sources of material and energy. What if an organism’s metabolism could be extended, within its lifetime, through interactions with other species? This “acquired metabolism” may be obtained through horizontal gene transfer, the theft of cellular machinery, or the formation of metabolic mutualisms. Such acquisitions enable phenotypic plasticity in the acquiring organism, allowing expansion of its fundamental metabolic niche, with implications for community dynamics and ecosystem function. In this talk, I focus on the acquisition of photosynthesis as a model for how acquired metabolism allows an organism to span trophic levels and function as a keystone regulator of ecosystem dynamics. In particular, I highlight recent work on the marine ciliate genus Mesodinium, whose members steal chloroplasts—and, in some cases, transcriptionally active nuclei—from their prey. Within this genus, some species are obligately phototrophic, relying on stolen photosynthetic machinery for almost all of their energetic needs yet nonetheless achieving tremendous ecological success and forming extensive red-water blooms, while others are entirely heterotrophic. This gradient of reliance on acquired metabolism provides insights into the fitness benefits of achieving phenotypic plasticity through acquired metabolism.
Ben Parker
What mechanisms generate genetic variation in phenotypic plasticity? Ecology and evolutionary genetics of the pea aphid wing polyphenism

Abstract
Phenotypically plastic responses to environmental cues exhibit genetic variation. Virtually nothing is known, however, about the mechanisms that generate variation in phenotypic plasticity. We study a textbook example of plasticity in pea aphids, which are typically unwinged but produce winged offspring in response to crowding. Using a genome-wide association panel, we surveyed variation in the response of aphid genotypes to a crowding assay. Genotypes ranged from producing zero to nearly 100 percent winged offspring, and we identified 26 genetic markers significantly associated with this variation. Most of these markers fell within or close to genes, including receptors for dopamine, acetylcholine, and glutamate. These are exciting candidates for genes underlying variation in plasticity because recent work has shown that neurotransmitters acting upstream of the hormone ecdysone trigger the broad changes in gene expression that produce the two morphs. We are currently using gene expression assays and pharmacological experiments to validate these mechanisms. We are also looking at variation across incipient species of pea aphids, which we have found vary in wing plasticity. Determining the kinds of mechanisms that are the targets of selection on phenotypic plasticity will help us understand how plasticity evolves and how it contributes to adaptive evolution and diversification.
Clare Rittschof
Honey bee aggression and brain energy metabolism: understanding the temporal components of behavioral plasticity

Abstract
Behavior presents unique challenges to our understanding of phenotypic plasticity. A behavioral phenotype reflects the integration of myriad experiences with variable timescales of effect. The mechanisms that underlie temporal variation in experience-induced behavioral change bear on fundamental assumptions about individual capacity for plasticity, and the ecological factors that shape its evolution. In the honey bee (Apis mellifera), anti-predator aggression is socially regulated at many timescales. Alarm pheromone induces aggression rapidly, while the tendency to respond to aggressive cues increases (at a rate that depends on the social environment) as a bee ages. In both plasticity contexts, brain transcriptomics link high aggression to decreased oxidative phosphorylation (OX, the major mitochondrial energy-producing pathway), suggesting
a simple model in which high aggression is induced and maintained by shifts in transcription of brain metabolic genes. However, new mitochondrial data suggest complex interactions between transcription and physiology: bees exposed to alarm pheromone show a rapid decline in brain OX, while older bees have much higher levels of OX relative to younger bees. Thus, the temporal element of plasticity in aggression involves an interplay between mitochondrial and transcriptional plasticity. Both levels must be considered to determine how experiences influence individual baseline aggressive state.
Daniel Schwab
Developmental plasticity, symbiosis, and niche construction: insights from the study of Onthophagus beetles
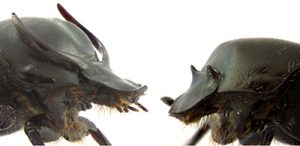
Abstract
Traditional perspectives on the ecology and evolution of developmental plasticity treat environments as being passive, external to, and separable from the organisms that experience them. Yet, environmental conditions internal to the organism (e.g. nutrition, hormones) can substantially bias the nature and outcomes of plastic responses, whereas external environments may themselves consist of other responsive and evolving organisms (e.g. developmental symbionts), and both internal and external environments can be shaped by the developing organisms themselves (e.g. via niche construction). Here, I use horn-polyphenic dung beetles in the genus Onthophagus to illustrate how integrating developmental symbiosis and niche construction enhances our understanding of the mechanisms and consequences of developmental plasticity. First, I discuss how serotonin and juvenile hormone signaling bias developmental outcomes and may have contributed to the diversification of horn polyphenism. Next, I show that the normative development of larvae is contingent on benefits (e.g. increased growth, developmental rate) derived from maternally inherited microbial symbionts. Intriguingly, these benefits appear to be highly context-dependent, shaping norms of reaction under ecologically-relevant stressors. Finally, I present emerging evidence that larvae behaviorally modify their developmental environment (i.e. niche construct), and show that these modifications generate environmental feedbacks that (i) shape plasticity in fitness-related traits and (ii) are necessary for the maintenance of sexual dimorphism. I end by discussing how the integration of internalistic and externalistic approaches offers a more holistic and complete account of plasticity and its evolution.
Laura Stein
The role of plasticity within and across generations in colonizing novel environments

Abstract
If phenotypic plasticity allows organisms to persist under new conditions, it may enable organisms to colonize and adapt to novel environments. I report a series of studies examining how plasticity in parents and subsequent effects on offspring might aid colonization of novel environments in two systems renowned for their rapid adaptation: threespine stickleback (Gasterosteus aculeatus) and Trinidadian guppies (Poecilia reticulata). First, I show evidence for adaptive transgenerational plasticity in response
to predation risk in both sticklebacks (paternal effects) and guppies (maternal effects). Second, I show how transgenerational plasticity might have contributed to the adaptive radiation of sticklebacks: there was greater variation in parental plasticity in ancestral compared to derived populations, with newly established populations intermediate. Third, I show that the brain gene expression profile of juvenile sticklebacks exposed to predation risk matched the brain gene expression profile of juveniles whose fathers were exposed to predation risk. These results are consistent with the hypothesis that developmental and transgenerational plasticity operate via a common set of molecular mechanisms.
Altogether, my results show that both mothers and fathers can transmit information and shape offspring development into adulthood, which suggests that transgenerational plasticity can play an important role in colonizing novel environments.
Maggie Wagner
Plasticity of constitutive glucosinolate content and implications for local adaptation in Boechera stricta

Abstract
Phenotypic plasticity is predicted to impact evolution by shifting values of traits that are under natural selection, but it is unclear how commonly, or under what conditions, plasticity moves traits in an “adaptive” direction. Progress on this question is currently limited by a lack of data from natural populations, and data on plasticity of physiological traits is particularly scarce. We conducted a large-scale field experiment to assess the extent to which glucosinolate defensive chemistry exhibits adaptive plasticity in the wild mustard Boechera stricta. We measured glucosinolate production and reproductive fitness for over 1,500 individuals comprising 25 known genotypes, in common gardens in four natural habitats. We detected extensive genetic variation for glucosinolate plasticity both within and among habitats. More often than expected by chance, plasticity moved glucosinolate profiles in the same direction favored by natural selection at each site. This finding suggests that glucosinolate plasticity tends to increase short-term fitness (relative to non-plastic B. stricta genotypes) upon colonization of new environments. Follow-up greenhouse experiments identified abiotic soil variation as one factor driving glucosinolate plasticity in these natural habitats. Additional work will be needed to assess the long-term impact of glucosinolate plasticity on local adaptation